Overcoming ESD-Control Flooring Challenges: A Comprehensive Guide to ANSI/ESD S20.20-2021
Navigating the Dissipative Versus Conductive Dilemma in ESD-Control Flooring Specifications

The demand for electrostatic discharge (ESD) flooring extends beyond the electronics manufacturing sector, encompassing call centers, data centers, and warehouses employing autonomous vehicles.
Photo: OCSiAl International.

The ultimate measure of success is not to achieve a certain resistance threshold across the floor but to prevent the accumulation of charge leading to a discharge of 100 volts or more from a person. This is how compliance with the standard is ultimately measured.
Photo: OCSiAl International.


The demand for electrostatic discharge (ESD) flooring extends beyond the electronics manufacturing sector, encompassing call centers, data centers, and warehouses employing autonomous vehicles. However, the industry faces challenges due to the complexity and risk of insulative spots associated with ESD-control flooring installations. ESD flooring specifiers often deal with a variety of standards and regulations, the most common of which is ANSI/ESD S20.20. Based on these standards, some confusion regarding the desired level of resistance for ESD-control floors appears. Due to this misunderstanding, specifiers may mandate resistance levels that, in the best case, add complexity and reduce aesthetic quality and, in the worst case, create risks to worker safety.
To overcome these hurdles, understanding of the ANSI/ESD S20.20-2021 standard and resistance levels is crucial.
What real level of resistance is necessary to protect electronic equipment?
The ANSI/ESD S20.20-2021 standard, developed by the EOS/ESD Association deals exclusively with the use of epoxy coatings as the ESD-control flooring material. Following are three things every specifier should understand about S20.20-2021 when specifying ESD-control flooring.
1) S20.20-2021 describes a program, not a flooring standard.
While ANSI/ESD S20.20-2021 plays a significant role in ESD-control facilities, it is essential to grasp that it outlines an ESD-control program encompassing various components, including flooring.
The stated purpose of the standard is to outline “the requirements necessary to design, establish, implement and maintain an ESD Control Program for activities that manufacture, process, assemble, install, package, label, service, test, inspect or otherwise handle electrical or electronic parts, assemblies and equipment susceptible to damage by electrostatic discharges greater than or equal to 100 volts Human Body Model (HBM).”
It’s important to keep this goal in mind when specifying flooring. The ultimate measure of success is not to achieve a certain resistance threshold across the floor but to prevent the accumulation of charge leading to a discharge of 100 volts or more from a person. This is how compliance with the standard is ultimately measured. Of course, the resistance to ground of the floor is essential in achieving this goal. The point is that floor resistance should be considered within this context. ANSI/ESD S20.20-2021 does this by specifying that the resistance of the flooring must be less than 1×10^9 Ω at all locations.
2) The distinction between dissipative and conductive is irrelevant .
Commonly, flooring within the 10^7 to 10^9 Ω range is considered dissipative, while flooring with resistance below 10^5 Ω is considered conductive. This has led to use of one megohm (1×10^6) as a convenient threshold for specifiers of ESD-control flooring.
However, properly installed flooring in the 10^7 to 10^9 Ω range can meet the charge accumulation requirements of S20.20-2021 while avoiding excessive conductivity risks. The key lies in aiming for the lower end of the static dissipative range and steering clear of the static conductive range. Specifying a firm one megohm threshold is unnecessary and counterproductive, as the focus should be on achieving resistance levels below 10^9 Ω, while also considering walking voltage tests.
3) More conductivity isn’t necessarily better.
The current interpretation of the standard often leads to one megohm resistance being widely specified. However, two scenarios can complicate matters. First, aiming for a precise target of 10^6 Ω can be challenging due to the logarithmic nature of resistivity and variations caused by temperature, humidity, and uneven dispersion of conductive materials.
That brings us to the second cause of believing lower resistance is inherently better. Again, the goal is to prevent accumulation of electrostatic charge on people and equipment leading to discharges of 100 volts or more. Considering again the logarithmic nature of resistivity that makes it difficult to hit a precise target, establishing a threshold of 10^5 Ω increases the likelihood the floor contributes to a shock hazard.
Rather than seeking to achieve the highest possible conductivity level, specifiers and building owners should be looking to reduce conductivity levels to the point where they can safely and consistently meet the S20.20 requirement of not discharging 100 volts. That level is resistance below 10^9 Ω.
How can you achieve conductivity with less material and zero insulative spots?
Traditional conductive materials like carbon black, tin oxide, and metal fibers often result in complicated installations, limited control over color and finish, the formation of hot spots, and the risk of an “orange peel” effect. The key reason is a loading rate that is generally in the 6% to 20% range.
A groundbreaking alternative emerges in the form of graphene nanotubes, such as TUBALLTM, produced by OCSiAl at scale. These nanotubes, also known as single wall carbon nanotubes, have a high aspect ratio and form an effective conductive network at very low loading rates, providing greater control over color and finish, and avoiding dark shades of the final coating. Incorporating just 0.01–0.05 wt.% of graphene nanotubes replaces higher percentages of other additives without compromising the formulation, leading to cost savings and a reduced environmental impact. Such floors can even be used without conductive primer.
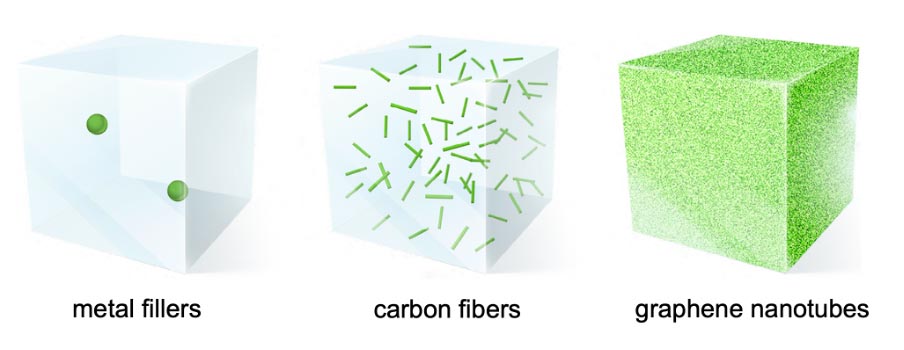
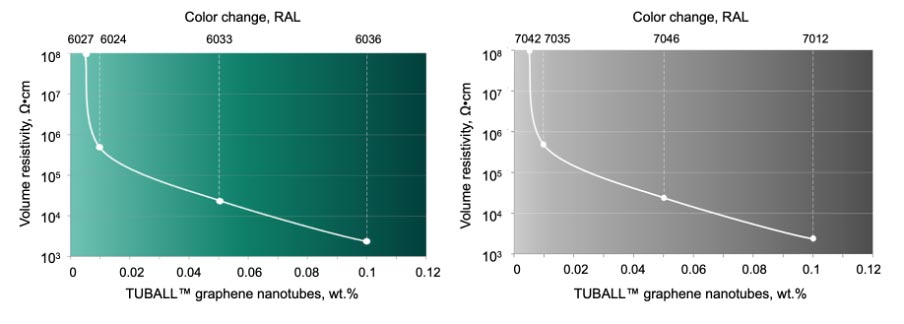
As the flooring industry embraces graphene nanotubes and specifiers gain a clearer understanding of the required resistance levels for S20.20-2021 compliance, many of the existing challenges fade away. Industries such as cleanrooms, pharmaceuticals, and automotive applications stand to benefit from these material innovations.
Looking for a reprint of this article?
From high-res PDFs to custom plaques, order your copy today!